Biomaterials must not only be approved as biocompatible but also specially developed for their intended use inside a medical device. This is crucial when considering the mechanical characteristics that determine how a particular biomaterial performs. The Young’s Modulus, E, which represents a material’s elastic response to stresses, is one of the most important material properties. For the best compatibility between the device and the body, whether the device is implanted or mounted externally, the Young’s Moduli of the tissue and the device that is being connected to it must nearly match. Ductility is crucial for implanted biomaterials that might be exposed to temperature changes, such as dental implants. For the same reason that the tensile strength cannot be excessive, the material must be ductile. Ductility allows the material to flex without breaking and also prevents the concentration of stresses in the tissue as the temperature changes. For dental implants as well as any other rigid, load-bearing implants, such a replacement hip joint, the material’s toughness is crucial.
Another crucial characteristic that needs to be taken into account for medical devices that are embedded or fastened to the skin is the flexural stiffness, D. Flexural rigidity will affect how effectively the device surface can maintain conformal contact with the tissue surface. This is crucial for devices that measure tissue motion (strain), electrical signals (impedance), or are made to adhere to the skin without delaminating, such as epidermal electronics.
The ultimate tensile strength of the biomaterial often decreases as its elasticity rises, and vice versa. Neural probes are one use where using a high-strength material is undesirable; in these applications, the tissue will always break before the device does (under applied load) since the dura mater and brain tissue have Young’s moduli on the order of 500 Pa. When this occurs, irreparable brain damage may result; as a result, the biomaterial must have a low tensile strength if an applied load is anticipated and an elastic modulus that is less than or equal to that of brain tissue.
By matching the elastic modulus, it is feasible to prevent stress concentrations that can cause mechanical failure and limit movement and delamination at the bio interface between the implant and tissue. Tensile and compressive strengths, which define the maximum stresses a material can bear before breaking, are other crucial characteristics. They can be used to set stress limitations that a device may be exposed to inside or outside the body. It may be advantageous for a biomaterial to have low strength in some applications, while it may be desirable for a biomaterial to have great strength in some applications, such that it is resistant to failure when subjected to a load.
Due to their innate flexibility and adaptable mechanical qualities, polymers are some of the most often utilized biocompatible materials (or biomaterials). A small number of plastics, such as cyclic olefin copolymer (COC), polycarbonate (PC), polyetherimide (PEI), medical-grade polyvinyl chloride (PVC), polyethersulfone (PES), polyethylene (PE), polyetheretherketone (PEEK), and even polypropylene, are frequently used to make medical equipment (PP). The use of biodegradable materials advances ethics while simultaneously enhancing the biocompatibility of implantable technologies. When evaluating various biodegradable biomaterials, a number of characteristics, including biocompatibility, are crucial. Depending on their source and kind of extracellular matrix, biodegradable biomaterials can be either artificial or natural (ECM).
High toughness enables biomaterial implants to endure longer inside the body, especially when subjected to enormous stress or cyclically loaded stressors, like the pressures imparted to a hip joint when running. Toughness characterizes the material’s capacity to deform under applied force without shattering.
Mechanical Properties of Biomaterials
on 22/06/2023
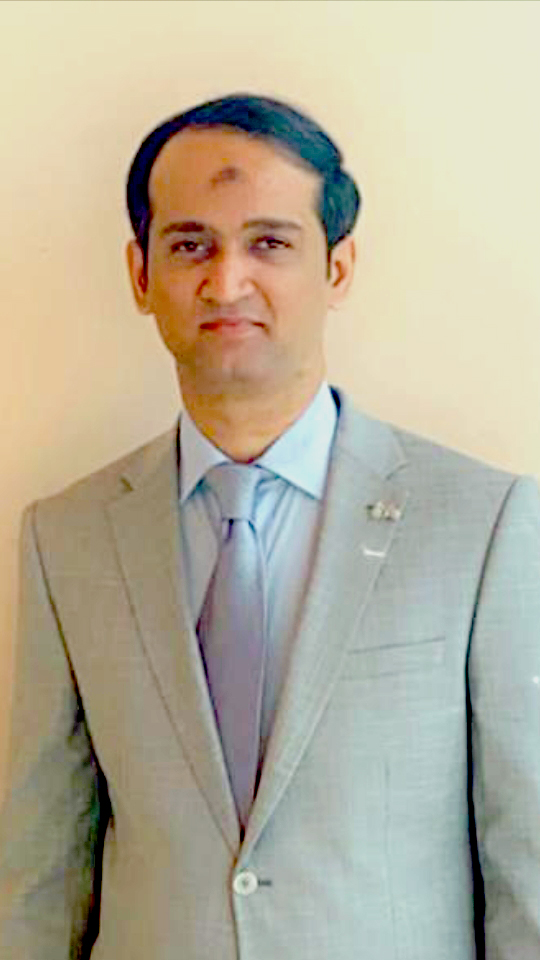